
The Financial Evaluation Structures of Cost-Optimal Building Renovation and Comparative Analysis of their Applications
ⓒ 2022. KIEAE all rights reserved.
Abstract
The cost-optimal solution for building remodeling is a crucial policy tackling the sluggish pace of greenhouse gas emission reduction in the building sector. Decision-making methods all stakeholders can rely on have been requested, and various methods have been suggested since the E.U.’s initial legal framework. This paper aims to analyze the structure of financial evaluations and their applications to establish the appropriate evaluation method in Korea.
This study analyzed cost-optimal calculation methods for building renovation adopted in recent research. Chronicle research trends, the basic structure of evaluation, the modified applications, and the reason behind the changes have been analyzed. Finally, the real risks for finding an optimal strategy based on the long-term feasibility analysis have also been addressed.
The trends regarding building renovation showed that the main drivers of building remodeling have altered from technical application to the feasibility of initial investment and flexible business models of staged renovation. The various applications of lifecycle cost for building renovation showed that 1)lifecycle evaluation requires supplementary info evaluating the adequacy of initial investment, 2) the feasibility evaluation by initial global costs did not show positive financial benefits, and the various modification frameworks have been adopted, and 3) reliability of initial cost data and long-term economic index needs to be set realistically. Stimulus methods to boost the viability of renovation within the calculation framework and the social agreement need to be researched further.
Keywords:
Building Renovation, Cost Optimal, Global Cost, Net Present Value키워드:
건물 리모델링, 비용최적화, 총비용, 순현재가치1. Introduction
The UNFCC confirmed scientific trust in climate change caused by global greenhouse gas emissions, and efforts to draw a global agreement for greenhouse gas reduction are continuing. Lifecycle greenhouse gas emissions from buildings account for a high proportion of national carbon dioxide emissions, so it has been a priority strategy in each country's greenhouse gas reduction policies. In particular, in many countries, where the urbanization stage has entered to completion stage and the volume of new building constructions compared to the total building volume is less than 1.5%[1], it is urgent to reduce greenhouse gas emissions by improving the energy efficiency of existing buildings. Therefore, in the 2000s, research on the application of remodeling technology and pilot projects were carried out worldwide, and each country introduced energy efficient building remodeling policy and is expected to achieve the goal of reducing greenhouse gas at a rapid pace.
E.U. state nations took the lead in implementing related policies. The U.K. established a policy to reduce carbon dioxide emissions by 29% in the housing sector between 2010 and 2020[2], and Germany introduced an energy-efficient building retrofit policy subsidies for investment and social policy of allowing landlords to increase the rent compensating their investment for energy efficient building renovation[3]. Similar policies are being carried out worldwide; Korea set a building energy renovation goal by 2030 of renovating 58% of old public buildings through the “Green Remodeling Mandatory Roadmap”. In the U.S., through the Architecture 2030 Challenge, the goal of achieving 90% of the energy use intensity (EUI) of existing buildings by 2025 and carbon-neutral (100%) by 2030 is being pursued[4]. Despite the policy change, challenging goals, and technology development, the achievements so far are not satisfactory. In order to achieve the E.U.'s current climate change migration goal, it concluded that two to three times more renovation works should be executed[5-6].
Despite the other obstacles, financial barriers have been the most significant constraint from the early phase of adopting building remodeling as a critical strategy for reducing greenhouse gas emissions[7-9]. Regulation No. 244/2012 was set into effect to tackle financial burdens in the market, and stipulates the cost-optimal analysis required for the selection of optimal technology for building renovation by law[10], as well as detailed procedures and standards for the analysis method. The reporting and utilization method have been introduced and follow-up research was carried out[11].
Since then, related research on evaluating the process of reestablishing policies using the financial evaluation methodology of cost-optimal decision-making and policies influenced by the research findings has been continuously evolving. The number of research papers that searched for cost, optimal, building, and renovation as the keywords were gradually increasing. Therefore, comparative research is required to establish an appropriate framework. Therefore, the purpose of this study is as follows(Fig. 1.) ; 1) the analysis of cost-optimal retrofit researches trends to derive major issues by period (chapter 2.), 2) the comparison of the cost-optimal evaluation methodologies and their application cases(chapter 3. and 4.), 3) major controversial issues which require continuous research in order to convert to a future trading system of carbon dioxide emission through the frameworks of building retrofits (chapter 5.).
2. Trends of Cost-Optimal Evaluation for Building Renovation
The conflicting mission to fulfill greenhouse gas emission reduction and financial viability of investment has brought a vast range of research regarding construction technologies, decision-making procedures, and financial viability evaluation has evolved so far. The standard in the early 2010s initiated by the E.U. stimulated the studies for cost-optimum building retrofit to derive a wide range of implementation methodologies; the regulation “(E.U.) No 244/2012”, which stipulates the cost-optimal methodology framework[10]. In the same era, the IEA project of “Cost-Effective Energy & CO2 Emissions Optimization in Building Renovation” was followed to aim for in-depth methodology and implementation tools. In particular, “shining examples” and “detailed case studies” has expanded the discussion of energy-efficient retrofit research[11]. Initially, the international energy agency (IEA) developed an optimal technology suitable for remodeling, and cost-optimal evaluation tools and decision-making processes followed. After that, “Business and Technical Concepts for Deep Energy Retrofit of Public Buildings (Annex 61, (2012 - 2017))” to establish and check a deep remodeling strategy to more aggressively achieve the greenhouse gas reduction goal while maintaining the cost-optimal strategy was carried out[12]. The iBRoad project (iBRoad project, 2018), which started in 2017, aims for profound remodeling methods to cut off carbon emissions[13].
The research trends of cost-optimal analysis methodologies for energy-efficient refurbishment after 2010 were divided into three phases; the initial methodology establishment phase with global cost, the mid-term phase of financial evaluation through net present value, and the later phase of deep renovation strategies. The summarized research contents of each phase are as follows;
2.1. Cost-optimum analysis with global cost assessment (2010~2014)
The overall methodology was established through multi-faceted researches including the building remodeling procedure, the design and construction technologies, the decision-making mechanism, and the economic evaluation methods[2]. Several analysis methods were introduced and tested despite the global cost method's legal framework.; payback period[14-15], delta LCC methods[16-18], global cost[19], and net present value[20] were utilized.
Research on the decision-making process for selecting priority among various technologies was conducted due to multi-faceted aspects of building refurbishment. Menassa and Bauer presented the house of quality model that could prioritize demands and technology applications in the project for various stakeholders[8].
2.2. Net present value evaluation (2015~2017)
Research has been conducted to clarify the financial investment value of building remodeling projects to support the owners' decision to undertake the project, identifying the investment value through the net present value. Net present value presents the economic benefits of projects by adding the concept of revenue to the concept of global cost and suggests the economic viability of projects more clearly.
NPV is defined as a cost-benefit evaluation framework through calculating the cash-in and cash-out flow during the lifecycle period. Since a virtual profit from the energy cost difference between the remodeled project and the existing one is the main component of cash-in flow, and the initial cost for remodeling is the main component of cash-out flow, the global cost difference can calculate the net present value after renovation. The global cost concept established in the previous period can be utilized. The detailed evaluation process of net present value and the global cost is described in chapter 3.
The various concepts of boosting the financial attractiveness of energy-efficient building refurbishment were introduced, including accounting for the anyway cost of an existing building and financial incentive programs. At first, the anyway renovation cost meant the minimum replacement and maintenance cost required to maintain the current level of energy performance[21], and the initial cost evaluation could be modified according to it. As a result, it escalates the initial cost of an existing building, reduces cash-out flow, and improves net present value, eventually. The financial benefits of grants, tax relief[22-24] and carbon allowance cost[25] also improve the investment attractiveness of remodeling projects were studied.
The non-finantial benefits of remodeling projects are presented owing to low financial return; improvemnet of various building quality and aesthetic or psychological benefits were raised[26-27]. Cost-optimization research also dealt with a social issue of owners' ability to invest. Diversified project financing was sought by invest capacity of building owners[28].
2.3. Deep remodeling and staged Implementation (2018~2021)
Concerns were raised about whether the greenhouse gas emission reduction goal could be achieved with cost-reasonable solutions, and an alternative deep remodeling strategy integrating cost rationality and energy-saving performance was simultaneously devised. Based on DIRECTIVE (EU) 2018/844, the concept of a building renovation passport, a long-term, step-by-step remodeling strategy for realizing a more aggressive reduction goal along with the goal of zero energy conversion by 2050 was established through deep renovation[29].
The building types for renovation have expanded to commercial offices and public schools considering diverse building programs in the real building stock[30-32]. The zero-emission target was also expanded to the long-term emission assessment, which included embodied energy for building materials[22].
3. Concepts and Structures of Cost–Benefit Analysis for Building Refurbishments
3.1. Life Cycle Cost Estimation: Global Cost
The concept of life cycle cost is essential for a green remodeling project because long-term virtual energy savings should be calculated as an exchange for current investments. The existing lifecycle cost analysis methodology was introduced in the initial phase of the cost-optimal remodeling methodology. However, E.U. regulation No. 244/2012 stipulated calculating the total cost during the life cycle period using primary energy consumption and initial investment cost, embracing the concept of lifecycle cost[10], and most studies have followed the global cost evaluation framework.
Global cost is the sum of the present value of all costs, including initial investment, operating and replacement costs, and disposal costs during the life cycle period. The initial investment cost is calculated at the current price, and the effect of expected future price developments is reflected in future costs such as energy cost development, the replacement cost of a specific part or equipment system, and disposal cost. In the end, the present values of each year's projected discount factor are summed up. The formula for calculating the global cost stipulated in Regulation No. 244/2012 is as follows (Eq. 1) and (Eq. 2).
(Eq. 1) |
where,
Ci : Initial investment costs for measures j
Ca,i(j) : Annual cost during year i for measures j
Vf,t(j) : Residual value of measures j at the end of the calculation period
Rd(i) : Discount factor for year i based on discount rate
t : Number of time periods
(Eq. 2) |
where,
Ce,i(j) : Annual energy cost during year i for measures j
Cm,i(j) : Annual maintenance cost during year i for measures j
Cdp,i(j) : Annual disposal cost during year i for measures j
t : Number of time periods
The concept of a remodeling project is a strategy wherein energy-saving benefits offset additional initial investment costs[33]; thus, selecting the lowest global cost alternative is the cost-optimal solution. The initial method has been modified to compare the global cost with the initial cost, choose an appropriate option with a premium cost enhancing the energy reducing rate, and present multiple alternatives on the Pareto front using multi-purpose optimization.
The reason for the development of various applications of global cost analysis is the complexity of the global cost index itself. Selecting the cost-optimum case still requires additional information to determine the initial investment cost and the ratio of initial investment cost to energy cost in global cost calculation. Fig. 2. showes the diffent usage of global cost evaluation. The left shows the methods to to select cases minimizing total global cost, but soverall global cost evaluation, but and the right depicts the additional information of cost compositions of construction, operating, and energy costs to convey the characteristics of alternatives in more detail.
3.2. Investment Decision Index : Net Present Value
The initial global cost study aimed at the relative evaluation of remodeling alternatives, which assumes a comparative evaluation of alternatives is conducted after confirming the implementation of the remodeling project. However, the initial global cost study did not provide various stakeholders with clear financial benefits of the remodeling project in advance. Thus, an alternative investment decision index evaluating costs and benefits was required.
The NPV analysis emphasizes the positive long-term benefits. The NPV method evaluates the sum of the present values of cash inflow and outflow during the life cycle of a building. From the perspective of cash flow in the general project investment, the investment cost is cash outflow (negative), and the present value of future energy saving is cash inflow (positive). If NPV is a negative value, meaningful financial profit creation or loss does not occur through the execution of the project, and the alternative can be determined to have no investment value. The NPV analysis was proposed as a decision-making indicator that can confirm the financial advantage of market participants when the renovation is conducted. The fundamental formula is presented in (Eq. 3).
(Eq. 3) |
where,
cash(In) :the cash inflow from the investment project (positive)
cash(Out): the cash outflow for initial investment and other operation cost (negative)
r : the real discount rate that could be earned in alternative investments
t : the number of periods
(Eq. 3) can be modified as (Eq. 4), reflecting the investment and financial benefits of the remodeling project. The annual energy saving in (Eq. 4) is the difference in energy cost between of an refurbished building and an existing one, thus it can be modified into (Eq. 5) reflecting the energy tarrifs. Then, the items can be rearranged with relation to the global cost method in (Eq. 6). Ultimately, the NPV can be calculated using the difference of global costs between an refurbished project and existing one shown in (Eq. 7).
(Eq. 4) |
where,
r : real discount rate (%)
N : lifespan, years
t : number of time periods
CR : initial refurbishment costs
Cm : maintenance cost
im : real growth rates of building construction, yearly (%)
SG, SO, SE : Annual saving of gas, oil, and electricity, respectively, yearly
ig, io, ie = real growth rates of gas, oil, and electricity, respectively, yearly (%)
Cdp : disposal cost of waste
Rv : residual value
The annual energy saving in (Eq. 4) is the difference in energy cost between of an refurbished building and an existing one, thus it can be modified into (Eq. 5) reflecting the energy tarrifs. Then, the items can be rearranged with relation to the global cost method in (Eq. 6). Ultimately, the NPV can be calculated using the difference of global costs between an refurbished project and existing one shown in (Eq. 7).
(Eq. 5) |
where :
CEG, CEO, CEE : Annual energy cost of gas, oil, and electricity for an existing building, respectively
CRG, CRO, CRE :the annual energy cost of gas, oil, and electricity for a refurbished building, respectively
(Eq. 6) |
(Eq. 7) |
where :
Cg Existing : global cost of reference building (Baseline)
Cg EER : global cost of energy-efficient refurbishment building (Project)
The positive NPV indicates that the global cost of the energy-efficient method is less than the global cost of the baseline model. Based on the NPV formula in (Eq. 5) and (Eq. 6), we can consider various strategies for improving NPV. Reduction of the generally recognized initial investment cost of EEP, widening the gap with existing building energy use through EEP, and maintenance through EEP. A relative reduction in cost and an increase in residual value through EEP can be considered.
The delta LCC is a concept similar to NPV, which evaluates the LCC of the remodeling project, compared with the reference model, during the life cycle. However, additional costs are indicated; thus, negative delta LCC indicates positive NPV. Fig. 3. shows the example application of delta LCC. Hamdy et al. suggested a preferable investment range and preferable cost-optimal solutions by comparing the application of energy-saving and renewable energy technology by calculating the LCC[17] and Ferrara et al. adopted the LCC analysis method and revealed that the improving envelope performance strategy has a more substantial effect on the cost-optimal solution than improving the equipment's efficiencies[34].
3.3 Long-term Economic Predictions
The discount factor is the converting ratio of future prices into current prices, and holds the same meaning as the interest rate in general, but the precise meaning is an index discounting the market interest rate (nominal interest rate) by reflecting the inflation rate. This is called the real discount rate or real interest rate. In majority of studies, a fixed discount rate was applied for NPV calculation without specifying the inflation and interest rates[35].
An approximation formula is sometimes used by subtracting the inflation rate from the nominal interest rate, which can be calculated using Equation (13-2). For example, the discount rate of 2.5% is applied in the study by Simson et al., wherein 3.5% nominal interest rate and 1% inflation rate is taken into account[36]. The impact of discount rate is clearly shown in the study by Ascione et al. (2017), wherein the higher discount rate resulted in a lower NPV because the main cash-in flow of energy savings in the future is more diminished in the higher discount rate case[37].
(Eq. 8) |
(Eq. 9) |
where,
r = real discount factor
i = market(nominal) interest rate
f = inflation rate
The energy bill mechanism is also considered carefully when estimating energy price, because the energy bill is not simplly proportional to energy consumption[38]. The installation cost, including installation of transformer and electric line, a minimum connection fee, and surcharge for the excessive use of energy, should be considered for the energy bill estimation.
4. The Application and Modifications of Financial Evaluation Methodology
The initial concept of GC and NPV has been supplemented by other indicators and modified to encompass the concerns of low financial benefits. Various applications represents some crucial considerations and common risks in the feasibility research. Table 1. presents the trends in critical issues related to cost-optimal methodology in the literature based on the period mentioned earlier: 1) the energy performance and energy cost of the reference building in EUI (kWh/m2a) and global cost per unit area (€/m2), 2) cost-optimal analysis period and applied interest rate. and 3) feasibility analysis characteristics.
Economic viability indicators used in each research analyzed comparatively based on following criteria;
1) whether anyway renovation was reflected in the baseline model cost calculation,
2) whether financial incentives were reflected, and
3) whether co-benefit and macro-economic analyses were performed.
The feasibility calculation methods are categorized into four types: annual energy saving, payback period(PBP), GC, NPV, and internal rate of return (IRR). The crucial research issues are summarized, and the financial performance of the alternatives is presented together with the energy and global cost per unit area, NPV of the alternatives, and PBP. The analysis period was 10–15 years for the PBP analysis; however, for most studies that applied the LCC concept, the building life cycle was applied as 30 years, with a few exceptions[39]. Most Analyses assumed the discount showed a 77% reduction, but the majority of energy saving was between 40% and 60%. The reduction rate of the global cost of the optimized case from the existing baseline model ranged between 11 and 56 percent.
4.1. Comparative Evaluation with Initial Investment
Despite the logical long-term financial evaluation methods, stakeholders in the real market, including building owners, are still willing to compare financial benefits proportional to the initial investment cost and expect a profit return in a short period. Thus, PBPs and IRR are used as auxiliary indicators for GC and NPV. PBP calculates the time until the sum of profit offsets the initial financial investment. In general, PBP is used for selecting single technology with a precise cost-benefit structure, such as a building element or HVAC system[40-42], but also used for the entire building project to supplement the LCC evaluation[43-45].
The standard PBP calculation method is a simplified cash flow model, which calculates the period from the end of a project. The standard PBP calculation method is a simplified cash flow model, which calculates the period from the end of project execution to the point at which the investment is recovered. The simple rate between 2% and 4%. The most prominent energy saving case payback calculates the benefits with the simple accumulation of expected annual profit that does not reflect inflation and interest rate. Therefore, there is a limitation in its use for an extended period[46]. A discounted payback period (DPP) indicator has been developed to overcome the simplified PBP method. The DPP evaluated by applying a discount rate for cash inflow, similar to the NPV method[39], is more appropriate. Although the DPP is an indicator for judging investment adequacy proportional to the initial investment, the IRR evaluates it in proportion to the real interest[42][47]. IRR is derived by calculating a specific discount rate at which NPV is zero, and a higher IRR indicates more financial attractiveness of an investment. Fig. 4. shows the difffence of optimized solutions in global cost calculation and payback period calculation[50]. The cases with higher initial cost and get high energy saving regults has a more attractiveness in the payback period calculation.
4.2. Improvement of NPV and non-financial benefits of Building Remodeling
Fig. 5. shows the NPV breakdown of remodeling projects with initial cost and the net present value of future energy-saving. The NPV calculations in the pilot projects revealed that the negative values could occur despite the energy savings by energy-efficient measures. The NPV also inherited a drawback complexity of the calculations and needs supplementary info.
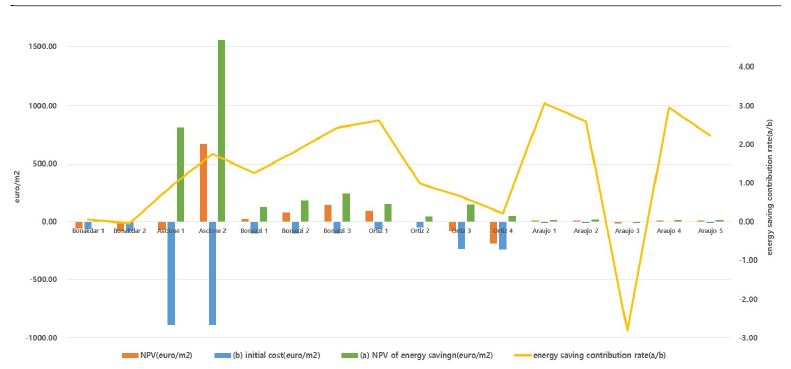
Comparison of initial cost, NPV of energy savings with remodeling, NPV of remodeling projects, and energy saving contribution rates in cost-optimal researches
In the fundamental NPV calculation method, a practical question has been addressed regarding whether the existing building, the reference building, will not undergo any construction work during the subsequent building life cycle. Consequently, the concept of anyway remodeling cost has been raised; this is the required cost for maintaining the reference building in the existing condition without initial investment for high remodeling cost[27][47]. The anyway remodeling cost can have a crucial impact on NPV results because it leads to some deduction of investment cost. When the anyway cost is reflected as the maintenance cost of the existing building, however, clear standards for calculating the anyway renovation cost have yet to be developed, and defining the scope of the maintenance construction is hard to implement, because the works scopes can vary from minor works, such as repainting to the replacement of windows and the entire wall[21][49]. Therefore, different approaches has been developed for assessing the anyway remodeling cost. Kumbarolu et al. suggested a case of fulfilling the minimum legal standard as the realistic anyway renovation cost calculation and Wrålsen et al. calculated the minimum cost for performing value-preserving in detail[31][51].
The public incentive for building renovation is a crucial factor in overcoming financial barriers noted in several market survey reports[52-53]. There have been wide-spreading worries regarding public incentives, such as incentive-free riders[54], an increase in product prices owing to demands increase triggered by subsidies[55], and social inequity among beneficiaries[56]. However, the need for public incentives is further significant when building retrofit targets shift from cost-optimal to high-performance levels.
The current incentive system for building renovation has various types of grants, tax relief, and subsidies for loan repayment. Thus far, it is generalized to support specific technical items, such as the installation of insulation and windows [22-24], but it is gradually transforming into a performance-based system[57]. The performance-based incentive systems are a cost-effective policy to guarantee the reduction of carbon emissions because a study[54] has shown that the same performance improvement package resulted in differences in energy savings more than five times according to the building completion year. The carbon tax relief for energy-efficient buildings also takes advantage of the performance-based incentive system, which imposes tax according to the energy savings and carbon emission reductions[58].
The type of subsidy also affects the financial analysis differently. The lump-sum payment method deducts the initial investment cost for subsidies like grants. In the case of tax relief and energy-saving-linked subsidies, the annual cash flow formula should be modified. The long-term cash-out flow of loan repayment and cash-in flow of tax relief can dilute the impact of the long-term economic index. The fundamental precondition for the public incentive program is the establishment of social infrastructures of information platforms, including financial and technical guidelines, which are available in the region[59], which can evolve into a private-public partnership[60]. In the case of the performance-based incentive system, the performance auditing and monitoring system should be prepared[2].
The revitalization of the carbon credit market in the building sector that each government adopted for utilizing the market mechanism is being discussed under the concept of "Effective and Socially Just EU ETS 2"[61]. The spontaneous participation in high-performance buildings has not operated thus far, even if EC has already applied the carbon trade market in Annex-I of the "cost-optimal methodology framework[10]. According to the principle of paying the person responsible for the carbon emission, the EU plans to endow CO2 emission allowances to the calculated carbon emissions. The initial cost would be €35 until 2030 for unit carbon emission and would increase to €50 after 2030.
Furthermore, the final goal of the zero-emission target also needs the cradle-to-grave concept. Ramírez-Villegas et al. proposed a calculation method of embodied carbon emissions by establishing the carbon emission inventory for construction materials[62]. In contrast, Debacker et al. presented a relatively indirect method of assessing impacts on humans and the ecosystem by depleting resources based on statistics without a propound inventory database[63].
As pilot projects implemented under the Energy in Buildings and Communities Programme of the International Energy Agency, non-financial benefits have been highlighted even though they are not directly countable economically. The criteria of thermal comfort, quality of natural lighting, air quality, building physics, internal nose control, external noise control, aesthetics, safety, pride, and constructability were presented.
Among non-financial benefits, indoor thermal comfort can be quantitatively evaluated; thus, it was also used as a constraint of optimization along with minimizing energy consumption and cost. Mostavi et al. evaluated PMV and cloth surface temperature[64], and Ascione et al. evaluated overheating hours[65]. In comparison, the common usage of non-financial benefits is related to overall project strategy and post-occupancy evaluation by selecting priority measures on the project. W Ott et al. suggested a positive/negative impact matrix[27], and Almeida and Ferreira suggested a method of a three-point scale evaluating framework[46].
Owing to the difficulties to evaluate the overall benefits building owners are willing to pay, estimating the integrated benefits indirectly by configuring the escalation of estate transaction prices is suggested theoretically[66]. However, further reliable statistics should be collected to evaluate the overall estate price change by building green renovation.
5. Vulnerability of long-term financial Evaluation
The framework of cost-optimal analysis with GC and NPV gained legal status in the 2010s. However, the application of NPV relies on statistics and long-term prospects, so finding cost-optimal solutions is sensitive to both data.
5.1. Reliability of estimating initial cost
To account for the NPV difference between existing and renovated projects, the significant cash-in and cash-out factors for deriving NPVs are initial investment cost and energy saving. Most research has focused on seeking cost-optimal solutions and various optimization methods, but the reliability of the cost-performance evaluation is highly dependent on fundamental market price statistics.
At first, EC emphasized the valid market-based cost at the time of analysis, and recommended member states to establish reliable construction cost database[10]. The trustworthiness of the cost data was acknowledged as one of the most challenging barriers to the early implementation of cost-optimal renovation but source information for initial cost data was not provided in the early researches. However, later, the country and regional level construction material databases were accurately specified. Data sources used in the research take advantage of public and private statistics of the quantity surveying specialists or associations[25, 67-68]or public bidding data[48][69].
Cost data from local market price surveys[23] are also used as supplementary data in the cost database. Even if the cost data were established, the fundamental difficulty remains. Various products are available in the market with the same performance because the product's market price is also related to other qualifications, including durability, warranty, and brand value[70].
During the early stage of the optimization study, the prototype method would calculate the cost by assessing the change of insulating materials, which are proportional to the thickness variation. The difficulty in estimating the initial cost of refurbishment is the various existing conditions. Furthermore, the refurbishment also requires a combination of various qualities, including airtightness, thermal bridge-free design, durability, constructability, and even aesthetics. The propound cost estimating method is required to reflect the refurbishment plan in reality. The cost data structure needs to be composed of three subcategories, including material costs, labor costs, and indirect costs, as Pombo et al. suggested[68] and the cost estimation includes all construction layers, including finishing material, airtightness tapes, waterproof membranes, and interior materials, as Simson et al. proposed[69]. The long-term cost change can be traced in detail to parameterize each item. In addition, cost estimation for renovation works should include the cost of partial dismantling, cleaning, and ground treatment, which are not likely to occur in new constructions.
Finally, the cost database for remodeling is insufficient because of the lack of relevant cases completed. Therefore, long-term data collecting for various building types, building systems, and years of construction should be established, as Lohse et al. recommended[48].
5.2. Long-term economic index
Several studies have revealed that energy price development and discount factors, which are indicators that convert future energy savings into present values, significantly impact NPV calculation. Several studies proved that discount factors and energy price development are more sensitive to NPV than initial cost and energy savings. Malatji et al. and Amstalden et al. demonstrated energy price development as the most critical factor compared to all other factors, including initial building refurbishment costs[40][58]. In contrast, Moore et al.‘s research showed that the discount factor has the highest sensitivity[71].
Many studies have applied the 3% discount rate applied in the studies by Ferrara et al. and Mostavi et al.[37][64], as recommended by the Commission Delegated Regulation (EU) No. 244/2012 (EC, 2012). In contrast, some other studies used a comparatively high discount rate of 5% and 8%[67][72] . Since the discount rate significantly affects the NPV, a realistic indicator must be set in consideration of the regional economic and social conditions.
The most notable effect of both the discount rate and energy price development is their conflicting aspect—the higher energy price development results in more positive values of NPV calculation. Suppose energy price escalation exceeds the discounting interest rate. The present value of the energy bill in a specific year becomes higher than the energy bill at the beginning of the project. For example, if the rate of increase in energy cost is 3% and the discount rate is 2%, in reality, the effect of increasing the energy price is 1%. Fig. 6. illustrated the range of discount rates in EU states in the cost-optimal researches and the counter –relashionship between discount rate and energy price development. Therefore, when the energy price development rate exceeds the discounting, the initial investment can be compensated by energy savings. The projects become more financially attractive, as shown in Kurnitski et al.[73].
6. Conclusion
Improving energy efficiency in the existing building stock can reduce greenhouse gas emissions. Efforts to seek cost-optimal alternatives led to significant research because the financial barriers had been revealed as the primary obstacle to building remodeling with energy-efficient measures.
The study analyzed cost-optimal concept and their applications in four phases. The trend of cost-optimal research was analyzed, and the actual global cost and NPV concepts and their application were analyzed comparatively. Af last, the vulnerability of cost-optimal research.
With the market’s demand for relevant financial information and the benefits of building refurbishment, the global cost concept has been adopted. The NPV was proposed as a more apparent financial decision-making tool. However, several pilot projects showed that the cost-optimal strategy is insufficient to achieve the carbon reduction target, so the deep and staged remodeling strategy has been suggested.
The researchers suggested various modifications of cost-optimal analysis. The coparative evaluations with initial costs such as payback period and interal rate of return were presented as supplementary indicators. The critical modification is the attempt to enhance economic attractiveness of building remodeling. The anyway cost concept was adopted to reduce initial investment; public incentives were included in the calculation; the effects of carbon trade were suggested; the non-financial benefits were emphasized.
Even though the framework have been widely acknowledged, researches also revealed the vulnerability of the long-term cost evaluation method. At first, the reliability of the initial cost is crucial, and the reliability of the cost database and the cost items for refurbishment should be considered. The long-term economic index proved the most critical variable for the feasibiltiy analysis, so the realistic range of discount factor and energy price development rate should be applied.
This research has analyzed the fundamental structure, application, and controversial issues regarding the cost-effective alternative searching process. The bespoke case analysis, the reliable database for initial costs and long-term economic index should be researched in the future. The initial cost estimation based on the geometric modifications caused by window change and dismantling, additional construction works, and the NPV with the energy-saving contribution rate of various remodeling scenarios will be researched further based on this study.
Acknowledgments
This research is supported by the Korea Agency for Infrastructure Technology Advancement (KAIA) grant funded by the Ministry of Land, Infrastructure and Transport (Grant 22AUDP-C151639-04).
References
-
C. Baek,, S. Park, Policy measures to overcome barriers to energy renovation of existing buildings, Renewable and Sustainable Energy Reviews, 16(6), 2012, pp.3939-3947.
[https://doi.org/10.1016/j.rser.2012.03.046]
-
Z. Ma et al., Existing building retrofits: Methodology and state-of-the-art, Energy and Buildings. 55, 2012, pp.889–902.
[https://doi.org/10.1016/j.enbuild.2012.08.018]
-
G. Kumbaroglu, R. Madlener, Evaluation of economically optimal retrofit investment options for energy savings in buildings, Energy and Buildings, 49, 2012, pp.327–334.
[https://doi.org/10.1016/j.enbuild.2012.02.022]
- J. Thorne, Unlocking ultra-low energy performance in existing buildings, Amerian Council for an Energy Efficient Economy, 2017.
- European Commission, Questions and answers on the renovation wave, 2020.
- European Parliament, S&D position on the Renovation Wave strategy, 2020, pp.1–16.
- M. Jakob, The drivers of and barriers to energy efficiency in renovation decisions of single-family home-owners, CEPE Working Paper Serires, 18, 2017, pp.1–30.
-
C. Menassa, B. Baer, A framework to assess the role of stakeholders in sustainable building retrofit decisions, Sustainable Cities and Society, 10, 2014, pp.207–221.
[https://doi.org/10.1016/j.scs.2013.09.002]
- N. Meyer, B. Mathiesen, F. Hvelplund, Barriers and potential solutions for energy renovation of buildings in Denmark, International Journal of Sustainable Energy Planning and Management, 2014, pp.59-66.
- European Commission. Commission Delegated Regulation (EU) No 244/ 2012 of 16 January 2012 supplementing Directive 2010/31/EU of the European Parliament and of the Council on the energy performance of buildings by establishing a comparative methodology framework for calculating. Off. J. Eur. Union 28.
- International Energy Agency Energy in Buildings and Cmmunities Programme, Annex 56, http://www.iea-annex56.org, /, 2021.05.15.
- Energy in Buildings and Cmmunities Programme, Annex Projects, https://www.iea-ebc.org/projects/completed, , 2021.05.15.
- IBRoad. Μy Path Towards an Energy Efficient Home, https://ibroad-project.eu, /, 2021.05.15.
-
K. Droutsa et al., Ranking cost effective energy conservation measures for heating in Hellenic residential buildings, Energy and Buildings, 70, 2014, pp.318-332.
[https://doi.org/10.1016/j.enbuild.2013.11.029]
-
K. Perini, P. Rosasco, Cost-benefit analysis for green façades and living wall systems, Building and Environment, 70, 2013, pp.110-121.
[https://doi.org/10.1016/j.buildenv.2013.08.012]
-
M. Beccali et al., Energy retrofit of a single-family house: Life cycle net energy saving and environmental benefits, Renewable & Sustainable Energy Reviews, 27, 2013, pp.283-293.
[https://doi.org/10.1016/j.rser.2013.05.040]
-
M. Hamdy, A. Hasan, K. Siren, A multi-stage optimization method for cost-optimal and nearly-zero-energy building solutions in line with the EPBD-recast 2010, Energy and Buildings, 56, 2013, pp.189–203.
[https://doi.org/10.1016/j.enbuild.2012.08.023]
-
A. Mohamed et al., The performance of small scale multi-generation technologies in achieving cost-optimal and zero-energy office building solutions, Applied Energy, 152, 2015, pp.94–108.
[https://doi.org/10.1016/j.apenergy.2015.04.096]
-
E. Arumägi, T. Kalamees, Analysis of energy economic renovation for historic wooden apartment buildings in cold climates, Applied Energy, 115, 2014, pp.540–548.
[https://doi.org/10.1016/j.apenergy.2013.10.041]
-
E. Pikas, M. Thalfeldt, J. Kurnitski, Cost optimal and nearly zero energy building solutions for office buildings, Energy and Buildings, 74, 2014, pp.30–42.
[https://doi.org/10.1016/j.enbuild.2014.01.039]
-
O. Mørck et al., Shining examples analysed within the EBC Annex 56 project, Energy and Buildings, 127, 2016, pp.991–998.
[https://doi.org/10.1016/j.enbuild.2016.05.091]
-
T. Ashrafian, Z. Yilmaz, Z., N. Moazzen, A long-term strategy for energy and cost performance improvement of existing residential buildings: Step-by-step renovation in Turkey, E3S Web Conference, 2019, p.111.
[https://doi.org/10.1051/e3sconf/201911103040]
-
C. Carpino, R. Bruno, N. Arcuri, N. Social housing refurbishment in Mediterranean climate: Cost-optimal analysis towards the n-ZEB target, Energy and Buildings, 174, 2018, pp.642-656.
[https://doi.org/10.1016/j.enbuild.2018.06.052]
-
P. Penna et al., Multi-objectives optimization of Energy Efficiency Measures in existing buildings, Energy and Buildings, 95, 2015, pp.57–69.
[https://doi.org/10.1016/j.enbuild.2014.11.003]
-
V. Brandão et al., EPBD cost-optimal methodology: Application to the thermal rehabilitation of the building envelope of a Portuguese residential reference building, Energy and Buildings, 111, 2016, pp.12–25.
[https://doi.org/10.1016/j.enbuild.2015.11.006]
-
M. Ferreira, M. Almeida, A. Rodrigues, Impact of co-benefits on the assessment of energy related building renovation with a nearly-zero energy target, Energy and Buildings, 152, 2017, pp.587–601.
[https://doi.org/10.1016/j.enbuild.2017.07.066]
-
W. Ott, R. Bolliger, Pitfalls in the economic and ecological evaluation of energy related building renovation strategies and measures, Energy Procedia, 78, 2015, pp.2340–2345.
[https://doi.org/10.1016/j.egypro.2015.11.395]
-
A. Serrano-Jimenez, A. Barrios-Padura, M. Molina-Huelva, Towards a feasible strategy in Mediterranean building renovation through a multidisciplinary approach, Sustainable Cities and Society, 32, 2017, pp.532-546.
[https://doi.org/10.1016/j.scs.2017.05.002]
- European Commission. DIRECTIVE (EU) 2018/844 OF THE EUROPEAN PARLIAMENT AND OF THE COUNCIL of 30 May 2018 amending Directive 2010/31/EU on the energy performance of buildings and Directive 2012/27/EU on energy efficiency. 2018.
-
L.O. Uğur, N. Leblebici, An examination of the LEED green building certification system in terms of construction costs, Renewable and Sustainable Energy Reviews, 81, 2018, pp.1476–1483.
[https://doi.org/10.1016/j.rser.2017.05.210]
-
M. Hu, Optimal renovation strategies for education buildings-A novel BIM-BPM-BEM framework, Sustainability, 10(9), 2018, pp.3287-3308.
[https://doi.org/10.3390/su10093287]
-
Y. Fan, X. Xia, Energy-efficiency building retrofit planning for green building compliance, Building and Environment, 136, 2018, pp.312-321.
[https://doi.org/10.1016/j.buildenv.2018.03.044]
-
C. Baglivo et al., Cost-optimal analysis and technical comparison between standard and high efficient mono-residential buildings in a warm climate, Energy, 83, 2015, pp.560-575.
[https://doi.org/10.1016/j.energy.2015.02.062]
- T. Boermans et al., Cost optimal building performance requirements: Calculation methodology for reporting on national energy performance requirements on the basis of cost optimality within the framework of the EPBD, 2011, pp.1-38.
-
M. Ferrara et al., A simulation-based optimization method for cost-optimal analysis of nearly Zero Energy Buildings, Energy and Buildings, 84, 2014, pp.442–457.
[https://doi.org/10.1016/j.enbuild.2014.08.031]
-
R. Simson et al., Redefining cost-optimal nZEB levels for new residential buildings, E3S Web of Conferences, 111, 2019.
[https://doi.org/10.1051/e3sconf/201911103035]
-
F. Ascione et al., Resilience of robust cost-optimal energy retrofit of buildings to global warming: A multi-stage, multi-objective approach, Energy and Buildings, 153, 2017, pp.150–167.
[https://doi.org/10.1016/j.enbuild.2017.08.004]
-
G. Kumbaroglu, R. Madlener, Evaluation of economically optimal retrofit investment options for energy savings in buildings, Energy and Buildings, 49, 2012, pp.327–334.
[https://doi.org/10.1016/j.enbuild.2012.02.022]
-
G. Bonazzi, M. Iotti, Evaluation of investment in renovation to increase the quality of buildings: A Specific Discounted Cash Flow (DCF) Approach of Appraisal, Sustainability, 8(3), 2016, pp.268-285.
[https://doi.org/10.3390/su8030268]
-
E.M. Malatji, J. Zhang, X. Xia, A multiple objective optimisation model for building energy efficiency investment decision, Energy and Buildings, 61, 2013, pp.81–87.
[https://doi.org/10.1016/j.enbuild.2013.01.042]
-
M. Medineckiene, F. Björk, Owner preferences regarding renovation measures - The demonstration of using multi-criteria decision making, Journal of Civil Engineering and Management, 17, 2011, pp.284–295.
[https://doi.org/10.3846/13923730.2011.582380]
- T.S. Ong, C.H. Thum, Net present value and payback period for building integrated photovoltaic projects in Malaysia, International Journal of Academic Research in Business and Social Sciences, 3(2), 2013, pp.153-171.
-
J. Huang et al., Thermal properties optimization of envelope in energy-saving renovation of existing public buildings, Energy and Buildings, 75, 2014, pp.504–510.
[https://doi.org/10.1016/j.enbuild.2014.02.040]
-
M. Jradi, C.T. Veje, B.N. Jørgensen, A dynamic energy performance-driven approach for assessment of buildings energy Renovation–Danish case studies, Energy and Buildings, 158, 2018, pp.62–76.
[https://doi.org/10.1016/j.enbuild.2017.09.094]
-
J. Lizana et al., Multi-criteria assessment for the effective decision management in residential energy retrofitting, Energy and Buildings, 129, 2016, pp.284–307.
[https://doi.org/10.1016/j.enbuild.2016.07.043]
-
M. Almeida, M. Ferreira, Cost effective energy and carbon emissions optimization in building renovation (Annex 56), Energy and Buildings, 152, 2017, pp.718-738.
[https://doi.org/10.1016/j.enbuild.2017.07.050]
-
W. Yu et al., Application of multi-objective genetic algorithm to optimize energy efficiency and thermal comfort in building design, Energy and Buildings, 88, 2014, pp.135–143.
[https://doi.org/10.1016/j.enbuild.2014.11.063]
- R. Lohse, H. Staller, M. Riel, The economic challenges of deep energy renovation - Differences, similarities, and possible solutions in central Europe: Austria and Germany, ASHRAE Conference, 122, 2016, pp.69–87.
-
K. Mahapatra et al., Business models for full service energy renovation of single-family houses in Nordic countries, Applied Energy, 112, 2013, pp.1558–1565.
[https://doi.org/10.1016/j.apenergy.2013.01.010]
-
K. Kuusk, T. Kalamees, M. Maivel, Cost effectiveness of energy performance improvements in Estonian brick apartment buildings, Energy and Buildings, 77, 2014, pp.313-322.
[https://doi.org/10.1016/j.enbuild.2014.03.026]
-
B. Wrålsen, R. O’Born, C. Skaar, Life cycle assessment of an ambitious renovation of a Norwegian apartment building to nZEB standard, Energy and Buildings, 177, 2018, pp.197-206.
[https://doi.org/10.1016/j.enbuild.2018.07.036]
-
J. Weiss, E. Dunkelberg, T. Vogelpohl, Improving policy instruments to better tap into homeowner refurbishment potential: Lessons learned from a case study in Germany, Energy Policy, 44, 2012, pp.406-415.
[https://doi.org/10.1016/j.enpol.2012.02.006]
-
S. Karatasou, M. Laskari, M. Santamouris, Determinants of high electricity use and high energy consumption for space and water heating in European social housing: Socio-demographic and building characteristics, Energy and Buildings, 170, 2018, pp.107–114.
[https://doi.org/10.1016/j.enbuild.2018.04.019]
-
M. Jakob, Marginal Costs and co-benefits of energy efficiency investments, Energy Policy, 34, 2006, pp.172–187.
[https://doi.org/10.1016/j.enpol.2004.08.039]
-
Y. Chai et al., Incentive-based demand response model for maximizing benefits of electricity retailers, Journal of Modern Power Systems and Clean Energy, 7(6), 2019, pp.1644–1650.
[https://doi.org/10.1007/s40565-019-0504-y]
-
D. Streimikiene, T. Balezentis, Innovative policy schemes to promote renovation of multi-flat residential buildings and address the problems of energy poverty of aging societies in former socialist countries, Sustainability, 11, 2019, pp.2015-2032.
[https://doi.org/10.3390/su11072015]
-
B. Lee, The comparative study on incentive system for integrated design of low-energy buildings, Journal of Korea Academia-Industrial cooperation Society, 17, 2016, pp.129–139.
[https://doi.org/10.5762/KAIS.2016.17.6.129]
-
R. Amstalden et al., Economic potential of energy-efficient retrofitting in the Swiss residential building sector, Energy Policy, 35, 2007, pp.1819–1829.
[https://doi.org/10.1016/j.enpol.2006.05.018]
- H. Rescoop, G. Fitzgibbon, Report on drivers and barriers to the deployment of citizen-led renovation, REScoop EU, 2021.
-
Y. Xue, A. Temeljotov-Salaj, C.M. Lindkvist, Renovating the retrofit process: People-centered business models and co-created partnerships for low-energy buildings in Norway, Energy Research and Social Science, 85, 2022.
[https://doi.org/10.1016/j.erss.2021.102406]
- B. Held, C. Leisinger, Assessment of the EU Commission’s Proposal on an EU ETS for buildings & road transport (EU ETS 2) / Criteria for an effective and socially just EU, 2022, pp.1–48.
-
R. Ramírez-Villegas, O. Eriksson, T. Olofsson, Life cycle assessment of building renovation measures–trade-off between building materials and energy, Energies, 12(3), 2019, pp.344-359.
[https://doi.org/10.3390/en12030344]
-
W. Debacker et al., Identification of environmental and financial cost efficient heating and ventilation services for a typical residential building in Belgium, Journal of Cleaner Production, 57, 2013, pp.188–199.
[https://doi.org/10.1016/j.jclepro.2013.05.037]
-
E. Mostavi, S. Asadi, D. Boussaa, Development of a new methodology to optimize building life cycle cost, environmental impacts, and occupant satisfaction, Energy, 121, 2017, pp.606–615.
[https://doi.org/10.1016/j.energy.2017.01.049]
-
F. Ascione et al., Resilience of robust cost-optimal energy retrofit of buildings to global warming: A multi-stage, multi-objective approach, Energy and Buildings, 153, 2017, pp.150–167.
[https://doi.org/10.1016/j.enbuild.2017.08.004]
- Rocky Mountain Institute, How To calculate and present deep retrofit value, 2015, p.105.
-
V. Milić et al., Evaluation of energy renovation strategies for 12 historic building types using LCC optimization, Energy and Buildings, 197, 2019, pp.156–170.
[https://doi.org/10.1016/j.enbuild.2019.05.017]
-
O. Pombo et al., Sustainability assessment of energy saving measures: A multi-criteria approach for residential buildings retrofitting - A case study of the Spanish housing stock, Energy and Buildings, 116, 2016, pp.384–394.
[https://doi.org/10.1016/j.enbuild.2016.01.019]
-
R. Simson et al., Redefining cost-optimal nZEB levels for new residential buildings, E3S Web Conference, 111, 2019.
[https://doi.org/10.1051/e3sconf/201911103035]
- A. Engeling, T. Loga, Implementing the cost-optimal methodology in EU countries, Buildings Performance Institute Europe, 2016.
-
T. Moore, J. Morrissey, Lifecycle costing sensitivities for zero energy housing in Melbourne, Australia, Energy and Buildings, 79, 2014, pp.1-11.
[https://doi.org/10.1016/j.enbuild.2014.04.050]
-
L.O. Uğur, N. Leblebici, An examination of the LEED green building certification system in terms of construction costs, Renewable and Sustainable Energy Reviews, 81, 2018, pp.1476–1483.
[https://doi.org/10.1016/j.rser.2017.05.210]
-
J. Kurnitski et al., Cost optimal and nearly zero (nZEB) energy performance calculations for residential buildings with REHVA definition for nZEB national implementation, Energy and Buildings, 43, 2011, pp.3279–3288.
[https://doi.org/10.1016/j.enbuild.2011.08.033]