
Evaluation of the Natural Lighting Performance of Rooftop Daylight Installations for Multi-purpose Sports Hall in Seoul
ⓒ 2022. KIEAE all rights reserved.
Abstract
The demand for natural light to maintain studetns’ the physical and psychological health is increasingAs indoor activities are required in multi-purpose sports halls owing to the outbreak of Covid-19 and the worsening of outdoor fine dust pollution. Therefore, this study aimed to provide design information for architects and all stakeholders to rely on in the initial design stage.
This study analyzed useful daylight index and daylight glare probabilty for side-lit windows, rooftop lightwells, and hybrid types. This study examined daylight performance with the Radiance engine and compared the numerical results and patterns for cases with different window sizes to find optimized instances.
The side-lit clerestory and eye-level types showed the best daylight performance at window-wall-ratio of 20% and 15%, respectively. The sawtooth and the cone lightwell type proved superior performance among the rooftop types; they showed excellent useful daylight index performance in cases with window-floor-ratio of 10% or higher. The daylight glare probability results showed that in all subjective cases disturbing glare was not found; in particular, the rooftop application demonstrated well-controlled glare-free environments. Moreover, the hybrid type demonstrated significantly improved daylight performance compared with the basic separate applications. The best daylight performance was the hybrid cases of sawtooth and cone types with side-lit windows of window-wall-ratio 10%. This paper provides helpful information in the early design decision stages, and detailed design research is required in the future.
Keywords:
Daylight, Sports Hall, Useful Daylight Illumination, Daylight Glare Probability키워드:
자연채광, 다목적체육관, 가용자연채광지표, 자연채광현휘확율1. Introduction
Until the 1980s, school facilities in Korea underwent rapid quantitative expansion. The number of elementary schools decreased after peaking in 1990[1]. School closures are of significant concern, particularly in the old downtown area, where the number of students is rapidly declining. Accordingly, strategies to use school facilities with the local community are being proposed. The sports hall is a crucial facility that can mimic outdoor playgrounds for physical activity and serve as a multi-purpose space for local community use[2]. The co-beneficial strategy has continuously led to sports hall extensions, and the Covid-19 pandemic spread intensified the need for them. Most design competitions for multi-purpose sports halls require the active use of natural light. Still, the actual application of such cases is minimal owing to cost escalation and glare concerns compared with conventional sports hall design[3]. However, the main barrier to daylighting design is the lack of appropriate benefits and design information for educators, architects, and contractors. As a result, architects have rarely attempted to integrate natural lighting in their design proposals. Therefore, this study aims to present a guideline that provides information on the natural lighting design of multi-purpose sports halls. In detail, it aims to provide quantitative performance information to guide design decisions in the initial planning stage by providing comparative quantitative analysis and visual pattern information according to design alternatives. Both numerical and visual information helps select daylight design alternatives. The research flows are shown in Fig. 1. with literature review, simulation settings and structure of comparative evaluation. In addition, this study also aims to set the criteria for the area ratio satisfying the threshold ranges for the climate-based daylight modeling method (CBDM), which has the advantage of evaluating the overall daylight performance within large spaces.
2. Daylight Standard for Sports hall
2.1. Quantitative CBDM methods and standard for sports hall
The required illuminance level of artificial lighting for the school facility including sports hall is set as 150, 200, and 300lx for minimum, standard and maximum requirements[4]. In Europe, the UK CIBSE standard and European standard stipulate 300lx for sports halls in education facilities[5-6]. Professional sports halls and international sports facilities require a stricter standard of 500lx and 750lx, respectively. Currently, Korea's natural light evaluation standard of the daylight factor (DF) is stipulated in the G-SEED certification, a Korean eco-friendly building certification. However, DF, an analysis under a virtual fixed sky condition, differs from the actual experience of the daylighting environment of occupants. Unlike traditional static daylight performance indicators such as DF, CBDM is subject to ever-changing sky conditions and sun positions and can predict the actual daylight level for each building location[7]. Therefore, the use of CBDM using dynamic daytime performance indicators has recently been increasing throughout the architecture world[8-9].
CBDM has daylight autonomy (DA) and useful daylight illumination (UDI) indicators. DA calculates the ratio of hours exceeding the minimum illuminance during the working hours requiring illumination. For example, DA300/50% means that the requirement is to reach 300 lx at least for 50% of occupied hours. In contrast, UDI measures the ratio of hours within, above, and below the specified illuminance range through daylight over one year[7]. Unlike DA, UDI stipulates an appropriate maximum illuminance, so it excludes the period when the glare is perceptible from the calculation of appropriate natural lighting conditon. It represents reality more precisely.
The initial evaluation method proposed by Nabil and Mardaljevic was to analyze the ratio of time reaching the illuminance level between 100-2000lx at a simulation position during the occupied hours. Lindelöf and Morel suggested the revised version of UDI, in which the maximum threshold illuminance, 2000lx, was extended up to 3,000lx to enhance daylight harnessing potential. It became a typical illuminance range of UDI[10]. The lowest illuminance level is set at 100lx for the minimum UDI threshold. However, Mardaljevic, who suggested UDI for the first time, divided the UDI range into UDI-supplementary (100-300lx) and UDI-autonomous (300-3000lx)[11]. The UDI supplementary range means that lighting levels can be supplemented by artificial lighting with dimming control. Therefore, Education Funding Agency (EFA) recommended that the lower threshold level for the sports hall, which requires a high illuminance level and uniformity, can be modified up to 300lx[12].
In order to analyze the overall daylight performance in the space, the spatial calculation for DA and UDI are required. The spatial DA and UDI calculate the spatial ratio of sensing points reaching target illuminance level and above compared to the whole area. The LEED certification adopted sDA300/50% and requires at least 40% of sDA300/50% and average 55% for collecting certification marks[13] and EFA recommended at least 60% of sUDI60[12].
2.2. Glare standard for sports hall
Glare-free design is also critical in sports hall daylighting because multi-purpose sports halls require accurate space recognition and identification of moving objects, including opposite players and balls. Therefore, the poor quality of the visual environment can result in the degradation of the players' reaction speed[14]. Although the UDI index considers the prevention of glare by defining the maximum illuminance level, it helps cross-check the side effects through a direct glare analysis during daylighting design.
Since the glare index was developed based on subjective psychological ratings, numerical calculations have been challenging; thus, diverse evaluation methods have been developed and gradually evolved. For instance, the British glare index (BGI), daylight glare index, CIE glare index (CGI), unified glare rating system (UGR), and daylight glare probability (DGP) have been developed. BGI is an evaluation limited to point light sources such as artificial lighting that was developed by improving and correcting the evaluation of having a wide surface as a light source, such as a window in direct sunlight. CGI began evaluating glare based on the difference between the light source and the background illuminance of the entire view according to the viewing angle. The UGR was developed as an expanded and corrected index even with artificial lighting[15]. DGP evaluates multiple types of light sources simultaneously but uses the light source illuminance, the size of the azimuth angle within the field of view of the light source, the displacement at the center of the field of view, and the degree of adaptation according to the background illumination[16]. Ding adopted the uniformity value for glare evaluation instead of glare analysis[3]; few studies on glare in sports halls have been found. EN 12193 stipulates UGR, an artificial lighting standard; however, the dominant index has not been stipulated clearly.
3. Methodology
3.1. Daylighting types and design variables
The comparative performance simulation analysis was conducted for side-lit windows and rooftop light wells to establish daylight design guidelines for the sports hall. The analysis procedure follows below. At first, basic geometric window types with various design sets were established according to the window-to-wall ratio (WWR) and window-to-floor ratio (WFR), which significantly impact natural lighting performance. The daylighting performance simulation results were compared to select the most outperforming case. The overall spatial daylight performance results and daylighting patterns were obtained through radiance-based UDI analysis. The additional glare analysis was conducted and cross-checked the vulnerability of the intolerable discomfort conditions.
Then, the same daylighting simulation analysis procedure for the hybrid type, which has both side-lit window type and rooftop window type in combination, was analyzed. The shapes, dimensions, and WFR and WWR configuration for rooftop and hybrid installations are illustrated in Fig. 2. At first, this study analyzed the conventional side-lit window of WWR between 5-35% and the rooftop lightwell of WFR between 4-15%. The hybrid model was composed of a combination of side-lit and rooftop windows, which tried to find optimized side-lit windows for non-optimized rooftop windows.
For the rooftop light well type, linear and point types and two subtypes were selected; monitor and sawtooth types and cuboid and cone light well types. When it comes to the linear types, the monitor type has windows facing both north and south directions, such that it receives both direct southern light and diffuse northern light. However, the sawtooth type has an inclined reflection ceiling that scatters solar radiation through north-facing windows. The cuboid and cone lightwell types differ from the angle of the wall of the lightwells; the cuboid type has vertical reflecting walls, but the cone type has inclined walls. For each type, the unit lightwells were equally distributed, and Fig. 2. summarizes the dimensions of each unit. For the rooftop window types, 6-stage cases with WFR of between 4%–14% were analyzed, and for the hybrid window types, 2-stage underperformed cases are combined with 6-stage case s of side-lit windows with WWR of between 5%–30% on botheast and west facadces were analyzed.
The geometry of the sports hall was selected based on the prototype model of a longitudinal shape plan, with a length of approximately 33.3m, width of approximately 22.7m, and a height of 9m covering approximately 756m²as shown in Fig. 3.[17]. The prototype model can accommodate one basketball court or three badminton courts with ancillary facilities at both ends of the shorter sides, equivalent to approximately 17% of the area. The main classroom building tends to be placed in the east-west direction, which takes advantage of south-facing window thermal energy and daylight. The sports halls are commonly placed at right angles to the main building, and the longitudinal axis becomes the north-south axis.
3.2. Daylight simulation profiles
The daylight analysis module, Radiance, in the integrated environmental solutions virtual environment (IESVE), analyzed UDI and DGP with annual climate data of Seoul from the climate one building organization[18]. The simulation grid has 0.5m spacing which is located 1.5m above the floor. Based on previous literature research, the minimum and maximum illuminance threshold for UDI analysis were set to 300lx and 3000lx. The daily occupancy hours were from 9 am to 6 pm without a break. The UDI simulation has been presented as the area ratio of the area of autonomous UDI 60% and 80% to the total number of grid points. The DGP standard, cases under 0.35, means occupants cannot perceive the glare, so regular activities were possible. It was analyzed on the data of 22 September, the autumn equinox, at noon and 3 pm. Fig. 4. shows the view position and direction for the DGP analysis, assuming basketball and are the most frequent sports in multi-purpose sports halls. In the case of badminton, the player is located in the middle of the left service court and looks at a shuttlecock directly tothe net and 35 vertical degrees (view directions A and B). In the case of basketball, the player is located in the three-point line facing the rim directly and at a 45° angle with a vertical view angle of 50° (view directions C and D)[19-20].
The visual transmittance of windows was set at 0.7 in order to obtain sufficient visual light. Materials frequently used in Korean school sports hall facilities have been researched. Maple flooring, sound absorption wooden boards, and white painted metal panels were common materials for the floor, interior wall, and ceiling as interior finishing materials of sports halls in Korea. The range of visual light reflectance for woods on walls and floors was between 0.35 and 0.54, and 0.45 was applied. The typical white ceiling panel has visual transmittance of 0.83.
4. Results
4.1. UDI and DGP of side-lit windows
The side-lit window type cases demonstrated that the spatial ratio exceeding UDI of 60% and 80% (sUDI60 and sUDI80) peaked at 15% and 20%, respectively, and deteriorated as the WWR increased. Table 1. demonstrates that the maximum sUDI60 was 100% in the case of clerestory windows and 95.4% in the case of eye-level windows. As the WWR increased above the peak ratio, sUDI60 decreased. On the contrary, sUDI80 was 20% lower than UDI60 for clerestory window cases and 50% lower than sUDI60 for eye-level window cases.
Table 2. summarises the DGP in all side-lit window cases at viewing position and angles of A, B, C, and D at noon and 3 pm on the autumn equinox. The overall values did not exceed 35% and achieved the glare-free condition. However, the DGP values of clerestory windows of WWR 30% and 35% in A, B, and D view angles and clerestory windows above WWR 15% cases in D view angles at 3 pm had DGP values above 30%.
4.2. UDI and DGP of rooftop windows
The same simulations were conducted for rooftop windows as for side-lit windows. Table 3. shows the overall outperformance of the sawtooth and cone type in each linear and point type. The most notable performance was found in the cone type. The cuboid lightwell type demonstrated the worst performance.
The area ratio satisfying 60% or more of UDI (sUDI60) of cone type with WFR 10, 12, and 14% cases were 98.2, 99.5, and 99.8%, so daylight conditions are good above WFR 8%. The best cases of each type were the case of WFR 12% in monitor type, the case of WFR 12% in monitor type, the case of WFR 14% in cuboid type, and the case of WFR 14% in cone type. sUDI80% was very low for all types of rooftop windows due to limited inflow of direct sunlight.
Figs. 5. and 6. illustrate the UDI pattern of four types of rooftop windows with above 10% of WFR. In Fig. 5., the monitor type showed a wavy pattern owing to the mutual interference of direct and scattered light entering from the skylight in both directions. Conversely, the result of the sawtooth type showed a one-directional daylighting pattern with a semi-circular pattern around the south wall. In Fig. 6., the UDI patterns of the cuboid and cone types showed similar concentric circle-shaped patterns but a clear difference in performance, even though they had the same WFR. The glare analysis was conducted for two outperform types, the linear sawtooth and point cone type. Table 4. summarises the DGP values of the four viewing directions in the linear sawtooth type and point cone type cases. Both types showed much difference compared with the side-lit type window. The DGP values are far below the discomfort threshold of 0.35, so there will be no difficulty in physical activity. Fig. 7. shows the hemispherical image for DGP analysis at noon and 3 pm. In both cases of WFR 14%, the lighting source outside the lightwell was not found in the space except for the cone lightwell type at noon when the light can pass through the windows installed horizontally.
4.3. UDI of hybrid type windows
In previous chapter 4.2, the overall daylight performance of rooftop window type was promising. However, owing to concerns of cost increase, more feasible alternatives of combined installation of side-lit and rooftop types are also analyzed further. The cases with smaller WFR compared to the best cases in sawtooth and cone types, outperforming linear and point types, were selected, expecting the side-lit windows would supplement the insufficient daylights. The base model of sawtooth and cone type with WFR 6% and 8% were combined with a clerestory window and an eye-level window of WWR 5, 10, 15, 20, 25, and 30%.
Table 5. shows that the cases of WWR 15% in eye-level window combination and WWR 10% in clerestory window combination, both with sawtooth and cone types, achieved the best performance. In the clerestory windows combination, the best cases for sUDI60 and sUDI80 were optimized in cases of WWR 10%, reaching 100% sUDI60 in all combinations. In particular, sUDI80 of sawtooth type of WFR 6% and WFR 8% were 64.6% and 58.5%, surpassing those of base separate application of sawtooth base models. However, in eye-level windows combination, combinations of WWR 10% achieved the best sUDI60 results, and the combinations of WWR 15% revealed outperform in sUDI80 despite a slight decline of sUDI60. When comparing sawtooth and cone types, the sawtooth-based cases revealed better performance than the cone-based cases, proving to be more effective alternatives. The best sUDI80 results in the linear sawtooth model were 67.4, 72.0, 64.6, and 58.5 %, surpassing those in the point cone model of 32.7, 31.8, 9.7, and 11.0%.

Area ratio satisfying UDI targets of 60% and 80% according to their WFR in hybrid types of side window and rooftop window type cases
Figs. 8. and 9. show the UDI patterns of sawtooth-based cases. The sawtooth of WFR 6% with the eye-level window of WWR 15% case and WFR 8% with the eye-level window of WWR 15% case showed the most comfortable daylight conditions in terms of the quantitative UDI levels and the uniformity in the sports hall space. However, a little asymmetrical difference between the east and west sections of the sports hall was found in both best cases. The irregular patterns were found in all side-lit windows of WWR 10% cases, even if the sUDI60 reached the ultimate 100% level.
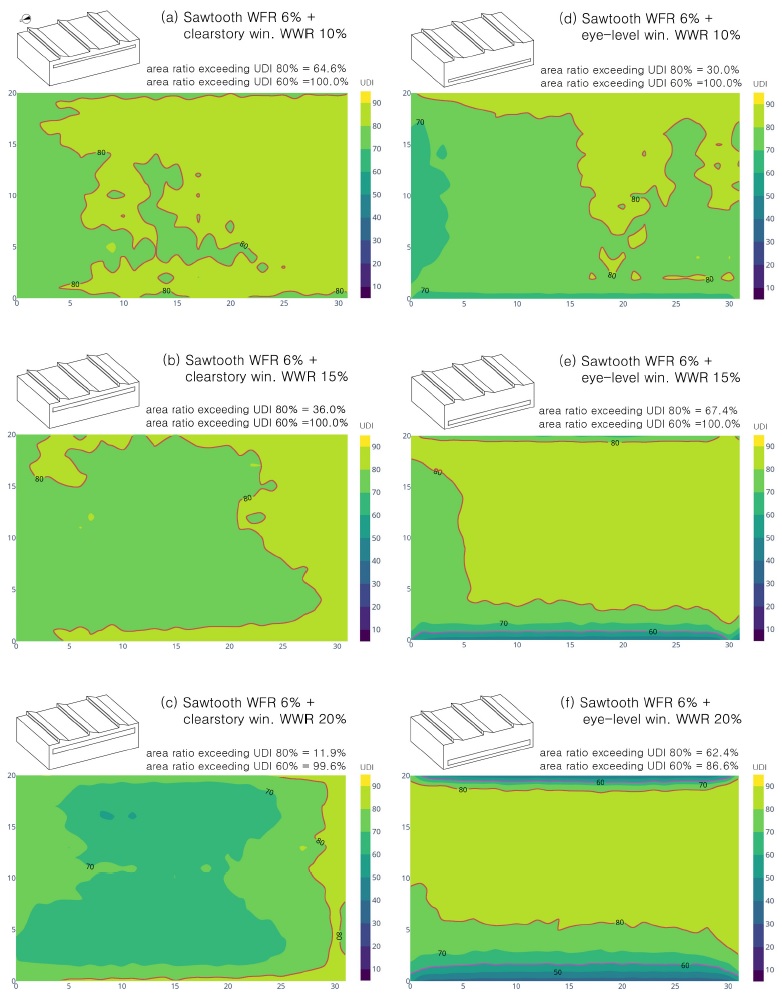
UDI patterns of sawtooth WFR 6% model based combinations with side-lit clerestory and eye-level windows
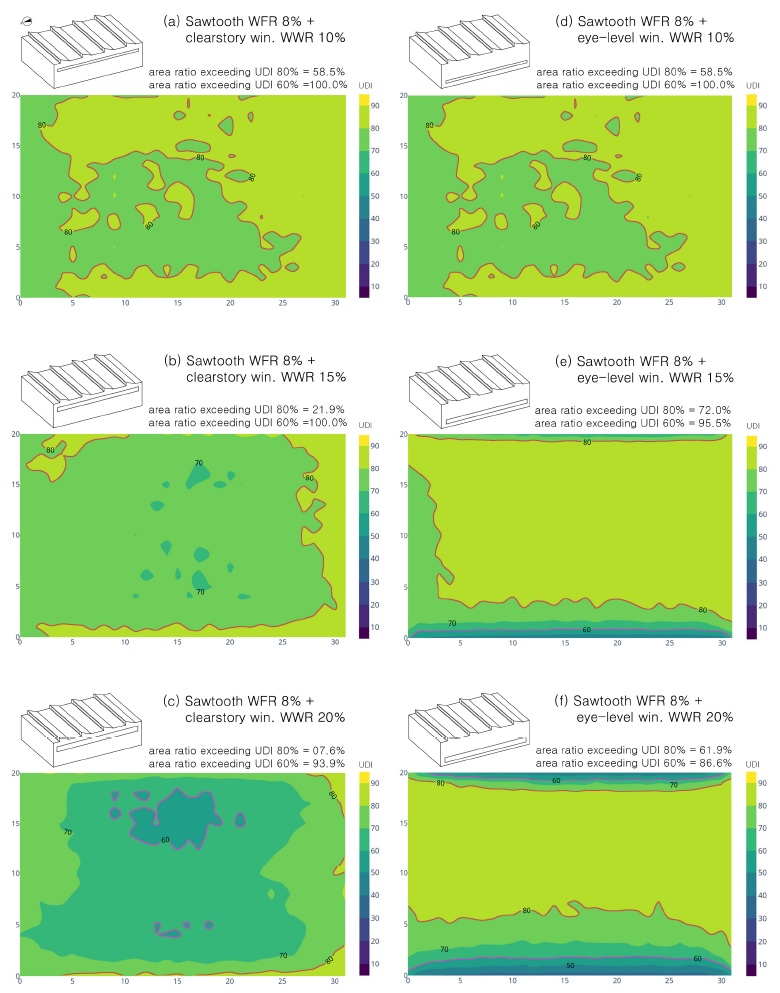
UDI patterns of sawtooth WFR 8% model based combinations with side-lit clerestory and eye-level windows
Figs. 10. and 11. show the UDI patterns of cone-based cases. Four cases of cone type of WFR 6% and 8% with clerestory and eye-level types WWR 15% demonstrated the most outstanding performance in the quantitative UDI levels. However, the combined cases of cone type with the eye-level window of WWR 15% had some irregular illuminance patterns, so the combination cases of cone type with the eye-level window of WWR 10% were proved to be preferable alternatives.
5. Discussion and Conclusion
This study aimed to provide daylight design guidelines for the initial design stage by comparing daylighting performance among common daylight types in multi-purpose sports halls. The side-lit, rooftop, and hybrid types with different window sizes were analyzed comparatively. Table 1., 3., and 5. show the daylight performance results and suggests the recommeded WFR and WWR range for each cases.
In the case of side-lit window types, the eye-level window and clerestory type, the cases of WWR 15% achieved the best UDI performance. It means installing large windows guarantees improving daylighting performance up to WWR 15%. However, for cases above WWR 15%, broad distributions of the UDI range deteriorated daylight performance and uniformity.
Fig. 12. represents the sUDI distribution of every sensing point in the rooftop window, and hybrid types. Fig. 12. (a)-(d), the UDI distribution of rooftop types showed the overall outperformance of sawtooth and cone types in linear and point rooftop types. The sawtooth and cone lightwell types of WFR 12% and 14% cases achieved peak UDI performances, respectively.
Fig. 12. (e)-(l) illustrate the UDI distribution of hybrid types, the combination of sawtooth and cone type of WFR of 6% and 8% with side-lit windows of WWR variations. The combination of side-lit of WWR 10% cases had the best performance considering UDI distribution's mean values and uniformity.
The DGP analysis for side-lit and rooftop cases demonstrated that the overall glare conditions were suitable for sports activity with DGP below 0.30, except for clerestory cases of WWR 30 and 35%. The DGP values in rooftop types did not surpass 0.25 and represented an excellent glare protection performance.
In this research, the spatial UDI calculating areas reaching 60% and 80% or more of the sports halls, the sUDI80 proved difficult for daylighting design. Further research for optimization studies on the detailed design for each type and the glare standard in an ample space is required.
References
- Statistics Korea, “Number of schools”, http://index.go.kr/potal/main/EachDtlPageDetail.do?idx_cd=1537, , 2022.03.15.
-
최보아, 최승호, 어린이 체험관 활성화 연구, 한국디자인포럼, 2007, pp.375–382.
B.A. Choi, S.H. Choi, A study on the promotion of children’s experience halls, Journal of Korea Design Forum, 2007, pp.375–382. [ https://doi.org/10.21326/ksdt.2007..16.036 ] - F. Ding, Daylight integration and visual comfort in sports halls in Norway, Master thesis, Norwegian University of Science and Technology, 2017.
-
국가기술표준원, 조도기준, 2018.
Korean Agency for Technology and Standards, Recommended levels of illuminace, 2018. - CIBSE:SLL, Lighting Guide 5-Lighting for Education, 2011.
- CSN EN 12193: Light and lighting - Sports lighting, 2018.
-
A. Nabil, J. Mardaljevic, Useful daylight illuminances: a replacement for daylight factors, Energy and Buildings, 2006, pp.905–913.
[https://doi.org/10.1016/j.enbuild.2006.03.013]
-
C. Reinhart, A model for manual and automated control of electric lighting and blinds, Solar Energy, 2004, pp.15–28.
[https://doi.org/10.1016/j.solener.2004.04.003]
-
V. Lo Verso et al., Questionnaires and simulations to assess day-lighting in Italian university classrooms for IEQ and energy issues, Energy and Buildings, 2021.
[https://doi.org/10.1016/j.enbuild.2021.111433]
-
D. Lindelöf, N. Morel, Bayesian estimation of visual discomfort, Building Research and Information, 2008, pp.83–96.
[https://doi.org/10.1080/09613210701544061]
- J. Mardaljevic et al., Daylighting metrics : is there a relation between useful daylight illuminance and daylight glare probability?, Proceedings of the Building Simulation and Optimization Conference BSO12, 2012.
- Education Funding Agency, EFA Daylight Design Guide, 2014, pp.1–15.
- USGBC, “LEED credit library”, https://www.usgbc.org/credits, , 2022. 05.15.
-
M. Pakkert et al., Glare quantification for indoor volleyball, Building and Environment, 2018, pp.48–58.
[https://doi.org/10.1016/j.buildenv.2018.06.053]
-
J. Wienold, J. Christoffersen, Evaluation methods and development of a new glare prediction model for daylight environments with the use of CCD cameras, Energy and Buildings, 2006, pp.743–757.
[https://doi.org/10.1016/j.enbuild.2006.03.017]
- J. Wienold, Daylight glare in offices, Fraunhofer ISE, 2009.
- W. Shin et al., The study about architectural plan and practical use of multipurpose room of school facilities, Review on the Korean Institute of Educational Facilities, 2004, pp.5–16.
- Climate One Builidng Organization, https://climate.onebuilding.org, , 2021. 05.15.
-
김선진 외 3인, 배드민턴 선수의 공격 방향 예측을 위한 시각탐색 전략과 반응 동작, 한국체육학회지, 제46권 제6호, 2007, pp.179–190.
S.J. Kim et al., Visual search strategies and reaction time differences between expert and intermediate badminton players, The Korean Journal of Physical Education, 46(6), 2007, pp.179–190. -
이동진, 정익수, 농구 3득점 점프슛 동작의 운동역학적 분석, 한국운동역학회지, 제20권 제1호, 2010, pp.49–55.
D.J. Lee, I.S. Jeong, Kinetic analysis of three-point jump shot in basketball, Korean Journal of Sport Biomechanics, 20(1), 2010, pp.49–55. [ https://doi.org/10.5103/KJSB.2010.20.1.049 ]