
Emergy-Simulation Based Building Retrofit
This paper introduces emergy(spelled with "m") that is a new environmental indicator in architecture, aiming to clarify conflicting claims of building design components in the process of energy-retrofit. Much of design practitioners' attention on low energy use in operational phases, may simply shift the lowered environmental impact within the building boundary to large consumption of energy in another area. Specifically, building energy reduction strategies without a holistic view starting from natural formation, may lead to the depletion of non-renewable geobiological sources (e.g. minerals, fossil fuels, etc.), which leaves a building with an isolated energy-efficient object. Therefore, to overcome the narrow outlook, this research discusses the total ecological impact of a building which embraces all process energy as well as environmental cost represented by emergy. A case study has been conducted to explore emergy-driven design work. In comparison with operational energy-driven scenarios, the results elucidate how energy and emergy-oriented decision-making bring about different design results, and quantify building components' emergy contribution in the end. An average-size (101.9 m2) single family house located in South Korea was sampled as a benchmark case, and the analysis of energy and material use was conducted for establishment of the baseline. Adoption of the small building is effective for the goal of study since this research intends to measure environmental impact according to variation of passive design elements (windows size, building orientation, wall materials) with new metric (emergy) regardless of mechanical systems. Performance simulations of operational energy were developed and analyzed separately from the calculation of emergy magnitudes in building construction, and then the total emergy demand of each proposed design was evaluated. Emergy synthesis results verify that the least operational energy scenario requires greater investment in indirect energy in construction, which clearly reveals that efficiency gains are likely to be overwhelmed by increment of material flows. This result places importance on consideration of indirect energy use underscoring necessity of emergy evaluation towards the environment-friendly building in broader sense.
Keywords:
Emergy, Building Emergy Synthesis, Building retrofit1. Introduction
1.1. Backgrounds
World Watch Institute reported buildings took up 40% global consumption of raw minerals and rocks as well as 55% of virgin wood each year. (Roodman and Lenssen, 1995). In the United States, the building sector accounts for the largest consumption of material and annual energy use (U.S. EIA, 2012). Accordingly, no longer should the notion of sustainability be simply a question of some of architects as built environment significantly changes our globe.
Recent growing attention on sustainability has been led to development of green building rating systems (e.g. LEED, Energy Star, CIBSE, etc.), and among many environmental indices, the dominant player is the operational energy performance that is estimated by building simulation or prescribed methods. In this case, adaptation of brand-new technology or enhancement of performing efficiency are the crucial matters to guarantee a system’s lower environmental impact.
However, as Willis (Braham, 2013) points out, characterization of building sustainability is not just limited to energy efficiency of a building, rather it should be understood as a macro phenomenon beyond the physical building boundary, since environmental impact is likely to be shifted from a building site to a neighborhood or even global societies (Zhang et al., 2010). Particularly for architectural practices, decisions in a building project, made by energy-driven procedures therefore occasionally result in higher construction costor being heedless of nonrenewable inputs (Malin, 2010).
In recognition of such problems, many studies to assess environmental loads in terms of both direct and indirect have already been attempted with various metrics. For example, Thormak (2002) analyzed a building's lifetime energy demand within the framework of life cycle analysis(LCA),and Pajchrowski et al. (2014) also characterized building energy use in aggregation with embodied energy of material flows. In addition, hybrid methods of LCA and embodied energy or exergy have recently been drawn upon to account for dynamic potential of sustainability in building design disciplines (Sakulpipatsin et al. 2010, Ramesh et al. 2010, Hernandez et al. 2010, Baek et al. 2013).
Nevertheless, as Srinivasan and Braham (2012) have pointed out, in the purview of right measurement on limited capacity of biosphere resources, above aggregation indicators leave much to be desired. It is because, even if those metrics were developed to pursue sustainability assessment in a larger scale, they fail to grasp the extensive boundary. To be specific, embodied energy does not consider quality of energy, and LCA begins with material extraction, not including initial formation process by natural work.
In this perspective, emergy (spelled with "m") pioneered by H.T. Odum, is a much more useful and can be a universal metric that offers a unique aggregate indicator scaling the most extensive impacts of building construction.
To explore emergy-driven assessment of building sustainability as well as clarification of competing sustainable design goals, it is obvious that designers have to keep track of the whole building retrofit process, and feeding it back for sufficient quantification.
However, as for building emergy analysis, unfortunately, fairly little research has been conducted. Instead, some case studies have attempted emergy evaluation on building manufacturing (Li et al. 2011, Meillaud et al. 2005, Pulselli et al. 2007), material recycling (Buranakarn 1998), or building envelopes (Pulselli et al. 2009, Price et al. 2010, Srinivasan et al. 2012). Meillaud et al. (2005) and Pulselli et al. (2007) carried out emergy analysis in accordance with building construction and maintenance. Srinivasan and Braham detailed long-term expectation of a building emergy with renewable inputs. Li et al. also utilized emergy indices to appraise ecological balance with respect to building manufacturing, but they only considered six or less materials which appear amiss to describe complex behavior of building construction. Schramski et al.(2008) and Pulselli et al. (2009) analyzed specific building envelopes (roof and wall) to gauge trade-off effects between energy improvement and compensating emergy variation. However, it reveals clear limit in that other major components acting towards the whole building analysis were not compiled into the study.
1.2. Objectives
Remainder of this research is to answer the following questions:
● Is it still valid to rate a building with operational energy performance estimation?
● What is ranked priority of building components among multiple design factors in light of the most extensive environmental impacts?
● Given a baseline model, how do we develop retrofitting scenarios with emergy indices?
2. Methods
2.1. Building Emergy Synthesis (BES)
Conception of emergy was first coined by H.T.Odum in early 1980s. As initiated from the study of cost benefits of energy and ecological environment, it has been developed to intend for modeling and evaluation of the work of systems of various scales in common metric. The idea of emergy centers on the assumption that systems of different types interact to work as being gained energy from one source-solar energy.
Analogy of all systems to biospherical networking allows to illustrate energy and materials, processed in a environmental system, through the holistic way of evaluation. Therefore, the use of emergy as an indicator of resource intensity has significant benefits since it reduces the various inputs and environmental services required for material cycles to a common denominator (Buranakarn, 1998).
Introduction of emergy analysis method is quite new to built environment as well as architecture. As an environmental accounting method, building emergy synthesis (BES) considers quantities of resources and quality of energy, which is termed as unit emergy value (UEV) or transformity. Calculating emergy indices of a building provides a new scale for evaluating environmental impacts by integrating energy use, material consumption, and other environmental services as represented by solar-emjoule (sej).
Solar-emjoule is a metric of emergy based on the principle that all embodied energy in both natural(e.g. water, rocks, etc.) and man-made products on the earth source from the solar inputs. 9.26×1024sej/yr is the baseline for all emergy accounting (Odum, 1996), and the measure of emergy is in units of the solar-emjoule.
H.T. Odum clearly illustrated dissimilarity in the behavior of energy and emergy with an example of typical basic configuration of a steady-state environmental system (Fig. 1.). In a typical ecosystem network, energy is always conserved from a source to a sink (Fig. 1(a)). However, emergy flows constantly through the chain in the same amount and does not be disrupted or disappear at the sink (Fig. 1(b)). This difference makes introduction of the notion of transformity useful to assess the quality of energy. In Fig. 1(c), numbers on the paths represent unit value of emergy. As the energy of feedback flow becomes the smallest (0.1J), transformity indicates the largest value (10,000 sej/J).
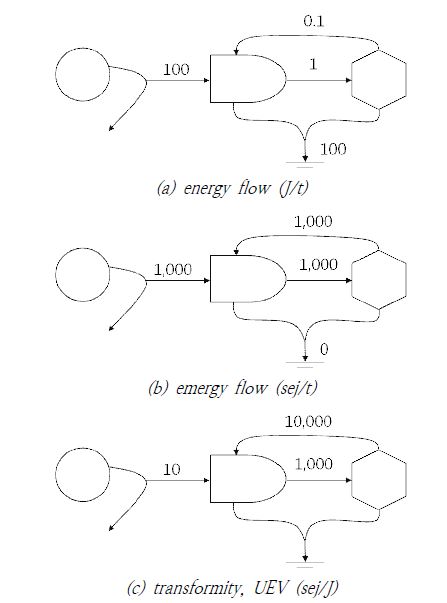
Concept of Emergy flow: This diagram comprises of four components; a circle stands for a source, a half-circular box is a producer of services, a hexagon is a consumer, and the rest is a sink (adapted from H.T. Odum, 1998).
For BES, emergy value(Em) of a building can be obtained as follows,
Emergy of materials is estimated by multiplication of its quantities and transformity. Accordingly, emergy demanded for manufacturing can be formulated by,
where ρ is unit weight of a material, V is volume, Tr is UEV of a material, and T is life time expectancy of a material. n is number of building components, and m is number of building materials.
2.2. Model description
To analyze retrofit decisions, a single-story building with common characteristics was attempted. The building under study is a real project pursuing green home certification but not yet built (Project named BK house, 386-8, YoungIn-city, South Korea). In order to provide generalized results, the sample was targeted to be a typical case of single-family house in South Korea. The site area is 474.0 m2, and building is oriented to the south. Floor area is 101.9 m2 which is approximately the average (102.3 m2) occupied by a four-strong Korean family. The building space is comprised of three bed rooms (9.1~13.2 m2) and a living room (19.3 m2) with a partitioned dining kitchen (19.8 m2). The structure is typical 2×6 light-weight wood frame with batt insulation infill (R-19, R-30). The split gable was adopted for roof (Fig. 2).
2.3. Baseline analysis
Based on the data sampled in the project, the baseline energy and emergy analysis were conducted respectively. For the whole building energy simulation, e-Quest 3.64 was utilized as recommended in ASHRAE Standard 90.1-2007. The space plan and design elements such as window size and location are client-dominated systems, but material properties affecting insulation should be guided.
Therefore, local building code of Energy-saving Design Guideline (Korea National Ministry of Land, 2012) was employed to set the base thermal conductivities such that, grad wall (150 mm) - 0.270W/m2K, roof (250mm) - 0.180W/m2K, ground floor - 0.230W/m2K, and glazing - 2.10W/m2K. Meanwhile, no specific regulation for operation schedule was found for the detached house. Accordingly, typical office daytime use planning was inputted: occupancy-08~19h, cooling- 13~17hour, and heating-09~19h for weekdays. For cooling, Split system single zone DX was implemented. Cooling coil make condensed air expand, and the electric motor supplies the cooled air into each room by a supply fan. On the other hand, thermal zones are heated by radiation produced by hot water coil in a boiler. Korean houses mostly adopt the radiant floor heating system, but US system-based eQuest does not provide the same equipment. Selection of an alternative system functioning similar is controversial thus far. Some argues that it can be modeled with low-temperature ventilator with zonal baseboard modules (Brahme et al., 2009), yet others find a solution in mimicking the system structure1). However, there is no such a rule set for temperature and specification of baseboard. Therefore, this study found the forced-air hot water heater with no ventilation approximates closest to this case, since radiation boards that hot water circulates work as terminal space heaters in the system, and no ventilation as well adds close analogy to the Korean heating method2).
Each demand of heating and cooling was calculated separately, and combined into total energy loads.
3D modeling in ECOTECT was used for material take-off, and having extracted quantities tabulated. For convenience of accounting, building emergy synthesis can be categorized into five different domains: structure, external skin, interior/ finishes, HVAC/electrical/ plumb system, and fixture/furniture /equipments. Each category is assumed to undergo the similar depreciation process and run out in exact life expectancy. External skin and structure, for instance, were assumed to last 50years, and 25years for interior /finishes. Input quantities multiplied by unit emergy values are summed within each category, and divided by presumed life cycle periods.
To estimate annual utility use, emergy synthesis is coupled with energy simulation results. The case study house is so simple and following local tradition that the experiment did not take airflow ducts or auxiliary ventilating machinery into account.
2.4. Proposed design
For comparative analysis, 11 passive-design items of interest are presented independently as variants, and each has three sub options according to presumed level of energy performance such as Good, Fair, and Baseline. Collection of 22 items is identified in the end for comparative evaluation as indicated in Table 3. As a decision-making level moves from Baseline to “Good”, it ensures the use of high-efficient building assemblies and lower operational energy consumption.
3. Results
3.1. Evaluation of Building Emergy in Baseline
Emergy calculation results obtained from the baseline is tabulated in Table 2. Table-form evaluations are added together to obtain total amount of emergy.
3.2. Emergy evaluation for proposed design
Table 4 summarizes the results of emergy evaluation of building manufacturing on each design item. According to the category to which it belongs, the amounts are scaled by their life time as a denominator. It varies from 5.9 × 104 sej/yr to 1.64 × 1014 sej/yr.
Combinations of each design item are incorporated into eight assemblages to come up with eight design scenarios as shown in Table 5. Metrics of natural gas use for heating (BTU) and electricity for cooling (kWh) were integrated into kWh for normalization. To convert energy consumption to emergy, UEVs of 4.00×104 sej/J and 2.69×105 sej/J are used for electricity and natural gas respectively. Note that transformity of electricity is higher than natural gas due to secondary processing to generate electricity from non-renewable and renewable resources. That is, using electricity for space conditioning deplete capacity of natural resources more. Considering that electricity is used for space cooling in residential buildings, how to reduce cooling demands can be more critical than heating. Total emergy of baseline is calculated as 9.85 × 1015 sej/yr. Design scenario 1 reinforced wall insulation, thereby saving operational energy to 9.6%. This, after all, led to the whole emergy improvement of 9.81 × 1015 sej/yr. Tightening up air leakages, in scenario 2, total emergy was reduced more without having any environmental loads from building manufacturing. However, 16% increment of energy efficiency in scenario 3 though, total emergy increased again to similar state as the baseline. It is due to the fact that energy saving did not compensate emergy rise from new material attachment involving various embodied energy consuming processes. Likewise, even though scenario 8 would be the best option in energy retrofitting of the building, it must not be the best in holistic perspective, since the total emergy value does not show the lowest. Such unbalance between energy driven retrofits and ones associated with BES is illustrated in Fig. 4. As though energy seems constantly decreased all over the scenarios, the emergy value of each alternative goes ups and downs without regard to energy efficiency.
To understand this results, investigation on each design component is inevitable. Table 4 shows total emergy value of components. (1) and (2) refers to level of energy performance. Plus sign means increment of total emergy. The most influential component is glazing type(G) regardless of energy performance level. For level (1), glazing increased emergy to 1.64 × 1014 sej/yr, and to 1.04 × 1014 sej/yr for level (2) in comparison with the baseline. Wall insulation(Iw) showed the largest difference between (1) and (2) (1.51 × 1014 and 0.60 × 1014 sej/yr). This result clearly demonstrates that energy reduction can benefit from high level of insulation, but over a certain point, the advantage may be directed to degradation of overall environmental soundness due to large increment of indirect energy required to produce high-performance insulation materials (Fig. 5).
It should be noted that change in ceiling height (Ch) is good for both operational energy and emergy. Reducing space volume benefits material flow reduction as well as increasing low-energy potential.
Fig. 5 describes contribution level of each design component in conjunction with total emergy values. The highest contribution level to emergy reducement is Ch for level (1), and the second is roof insulation(Ir) in level (2). Except for Ch, all others have reverse relations or even are irrelevant between energy and emergy. While blind installation (B) has little effect on construction emergy, it significantly affects operational energy use. Comparing wall insulation (Iw) and roof insulation (Ir), material energy inflow from high level of roof insulation is counterbalanced by low operational energy. Accordingly, one may notice that investment of high-performing roof takes advantage in terms of low environmental impact for the residential building.
4. Conclusions and discussion
This paper presents new environmental accounting methods employing emergy. Application to architectural practice with retrofit scenarios demonstrates its usefulness as a whole aggregation indicator.
In architecture and building industry, to reduce environmental resource consumption, technical measures are employed in processes, such as project decision-making and design (Li et al. 2011). Thus, design itself becomes a critical stage for comparative study on building components and various scenarios having competing actors. However, too often, questions on how to design a green building are simply targeted to encourage low-energy consumption or even zero-energy consumption.
Approaching high performance of operational energy only is a misconceived narrow perspective to make practitioners pursue well-designed technical systems, disdaining the ability of design that controls buildings' material flows in terms of which buildings are fully embedded in larger ecological contexts.
As proved in above, struggles to lower energy use are not identically effective to reduction of gross environmental impact. From the results, we have seen that higher energy-saving scenario may yield unexpected greater environmental impact against being environment-friendly by depleting biospherical energy resources more rapidly. This clearly indicates that design strategies for energy efficiency would not be reasonable decisions for achieving sustainability in genuine sense.
The results of this study confirms the argument that energy efficiency alone is not enough to design a truly environmental building which should impact as lowest as possible on nature from its birth to the end-of-life. In this light, that is, to prompt design process in closer associations with environment, why understandings of ecological impacts of built environment must be considered especially with the conception of emergy and reached practical application through emergy evaluations.
Briefly, emergy evaluation in design process offers two major strengths.
(1) It provides us with full insight of interaction between energy-saving and material changes through coupling of energy and mass/material flows.
(2) BES method models well-balanced and convincing approach with indicators of universal metrics in building construction and maintenance.
The test on a small-size building was enough to quantify covariance of emergy values corresponding to design variants, and yielded authentic results. Nevertheless, this study has limit in that the single sampling lacks certain parameters and connections with wider areas that a building comprises such as human activities, which might be led to more precise results. Detailed cradle-to-cradle analysis should also be followed to beef up the above argument. Describing larger buildings with wide breath of scenarios was out of time frame of this study, but such case can also be added to future considerations.
Glossary
1) See, http://www.warren-wilson.edu/~ELC/New_ELC_Website_/ radiant floorheat.php
2) Refer to the graphical representation of force-air hot water system at, http://visual.merriam-webster.com/house/ heating/forced-hot-water-system/forced-hot-water-system.php
References
-
C Baek, SH Park, M Suzuki, SH Lee, Life cycle carbon dioxide assessment tool for buildings in the schematic design phase, Energy and Buildings, (2013), 61, p275-287.
[https://doi.org/10.1016/j.enbuild.2013.01.025]
- WW Braham, Architecture and Energy Performance and Style, Florence:Routledge, (2013).
- R Brahme, Z O'Neill, W Sisson, K Otto, Using existing whole building energy tools for designing net-zero energy buildings - challenges and workarounds, Proceedings of the eleventh IBPSA Conference, (2009), p9-16.
- B Buranakarn, Evaluation of recycling and reuse of building materials using the emergy analysis method, University of Florida. PhD Thesis, (1998).
-
P Hernandez, P Kenny, From net energy to zero energy buildings: Defi ning life cycle zero energy buildings (LC-ZEB), Energy and Buildings, (2000), 42, p815-821.
[https://doi.org/10.1016/j.enbuild.2009.12.001]
- D Li, J Zhu, ECM Hui, BYP Leung, Q Li, An emergy analysis-based methodology for eco-efficiency evaluation of building manufac -turing, Ecological Indicators, (2011), 11, p1419-1425.
- N Malin, The Problem with Net-Zero Buildings (and the Case for Net-Zero Neighborhoods), BuildingGreen Inc 2010. (Available online at:http://www.buildinggreen.com/auth/article.cfm/2010/7/30/The-Problem-with-Net-Zero-Buildings-and-the-Case-for-Net-Zero-Neighborhoods/).
-
F Meillaud, JB Gay, MT Brown, Evaluation of a building using the emergy method, Solar Energy, (2005), 79, p204-212.
[https://doi.org/10.1016/j.solener.2004.11.003]
- HT Odum, Environmental Accounting: EMERGY and Decision Making, NewYork:John Wiley, (1996).
- G Pajchrowski, A Noskowiak, A Lewandowska, W Strykowski, Materials composition or energy characteristic―What is more important in environmental life cycle of buildings?, Building and Environment, (2014), 72, p15-27.
- JW Price, DR Tilley, Emergy Evaluation of a Green Façade, Proceedings from the Sixth Biennial Emergy Conference, (2010), p213-222.
- RM Pulselli, E Simoncini, FM Pulselli, S Bastianoni, Emergy analysis of building manufacturing, maintenance and use: Em-building indices to evaluate housing sustainability, Energy and Buildings, (2007), 39, p620-628.
-
RM Pulselli, E Simoncini, N Marchettini, Energy and emergy based cost-benefit evaluation of building envelops relative to geographical location and climate, Building and Environment, (2009), 44, p920-928.
[https://doi.org/10.1016/j.buildenv.2008.06.009]
-
T Ramesh, R Prakash, KK Shukla, Life cycle analysis of buildings: An overview, Energy and Buildings, (2010), 42, p1592-1600.
[https://doi.org/10.1016/j.enbuild.2010.05.007]
- DM Roodman, N. A Lenssen, Building Revolution: How Ecology and Health Concerns Are Transforming Construction, Worldwatch Paper, (1995), 124.
-
P Sakulpipatsina, LCM Itard, HJ Van der Kooi, EC Boelman, PG Luscuere, An exergy application for analysis of buildings and HVAC systems, Energy and Buildings, (2010), 42, p90-99.
[https://doi.org/10.1016/j.enbuild.2009.07.015]
- JR Schramski, DR Tilley, TL Carter, N Rustagi, Comparative Emergy Synthesis for Green Engineering, Proceedings from the Fifth Biennial Emergy Conference, p207-211.
- RS Srinivasan, WW Braham, DE Campbell, CD Curcija, Building envelope optimization using emergy analysis in environmental building design, Proceedings of the 12th International Building Performance Simulation Association Conference, (2011).
-
RS Srinivasan, WW Braham, DE Campbell, CD Curcija, Re(De) fining Net Zero Energy: Renewable Emergy Balance in environmental building design, Building and Environment, (2012), 47, p300-315.
[https://doi.org/10.1016/j.buildenv.2011.07.010]
-
C Thormark, A low energy building in a life-cycle-its embodied energy, energy need for operation and recycling potential, Building and Environment, (2002), 37, p429-435.
[https://doi.org/10.1016/S0360-1323(01)00033-6]
- U.S. EIA, Available online at: http://www.eia.gov/totalenergy/data/annual, (2012).
-
Y Zhang, S Singh, BR Bakshi, Accounting for Ecosystem Services in Life Cycle Assessment, Part I: A Critical Review, Environmental Science and Technology, (2010), 44(7), p2232-2242.
[https://doi.org/10.1021/es9021156]